Showing posts with label quantum mechanics. Show all posts
Showing posts with label quantum mechanics. Show all posts
Friday, November 22
Death Might be an Illusion
What happens after we die? While many believe that death is the end, quantum physics suggests that it might not be as simple as we think.
In fact, it could be an illusion. This idea challenges everything we know about life and death. By looking at concepts like the interconnectedness of all things and the nature of consciousness, here’s to a whole new perspective on life after death.
Dr. Robert Lanza, a leading expert in biotechnology, plays a major role in this idea. He’s the Chief Scientific Officer at the Astellas Institute for Regenerative Medicine, where he studies stem cells and how they can be used to treat diseases.
Before this, Dr. Lanza focused on researching embryonic stem cells and cloning, working with both animals and humans. He is also an adjunct professor at Wake Forest University School of Medicine in North Carolina. READ MORE...
Wednesday, November 13
Quantum Sensing
Researchers are exploring the potential to detect gravitons using quantum sensing technologies in hope of linking quantum mechanics with Einstein’s theory of relativity.
Advanced quantum sensing tools, such as those used at LIGO, detect gravitational waves by overcoming quantum noise through techniques like “squeezing.” These tools could also support graviton detection by providing a more precise way to measure gravitational disturbances in lab environments.
While technical and philosophical challenges remain, progress in quantum sensing may narrow the gap between quantum mechanics and gravitational theory, and provide new insights into phenomena like black holes and the Big Bang.
While each tasked with the important role of numerically explaining our reality, gravity and quantum mechanics tend to mix like oil and water — they have long presented a profound challenge to unify in the domain of physics. Albert Einstein’s general theory of relativity, established in 1915, describes gravity as the curvature of space-time. In contrast, quantum mechanics suggests that forces are mediated by particles. READ MORE...
While each tasked with the important role of numerically explaining our reality, gravity and quantum mechanics tend to mix like oil and water — they have long presented a profound challenge to unify in the domain of physics. Albert Einstein’s general theory of relativity, established in 1915, describes gravity as the curvature of space-time. In contrast, quantum mechanics suggests that forces are mediated by particles. READ MORE...
Saturday, October 19
Quantum AI Reshaping our World
In the ever-evolving landscape of technology, a new frontier is emerging that promises to reshape our world in ways we can scarcely imagine. This frontier is Quantum AI, the powerful fusion of quantum computing and artificial intelligence.
It's a field that's generating immense excitement and speculation across industries, from finance to healthcare, and it's not hard to see why. Quantum AI has the potential to solve complex problems at speeds that would make even our most advanced classical computers look like abacuses in comparison.
Demystifying Quantum AI: The Power Of Qubits And AI
But what exactly is Quantum AI, and why should you care? At its core, Quantum AI leverages the principles of quantum mechanics to process information in ways that classical computers simply can't.
Demystifying Quantum AI: The Power Of Qubits And AI
But what exactly is Quantum AI, and why should you care? At its core, Quantum AI leverages the principles of quantum mechanics to process information in ways that classical computers simply can't.
While traditional computers use bits that can be either 0 or 1, quantum computers use quantum bits or qubits, which can exist in multiple states simultaneously thanks to a phenomenon called superposition. This allows quantum computers to perform certain calculations exponentially faster than classical computers. READ MORE...
Tuesday, September 24
A Particle of Gravity Glimpsed
Gravitons, the particles thought to carry gravity, have never been seen in space – but something very similar has been detected in a semiconductor.
Physicists have been searching for gravitons, the hypothetical particles thought to carry gravity, for decades. These have never been detected in space, but graviton-like particles have now been seen in a semiconductor. Using these to understand gravitons’ behaviour could help unite the general theory of relativity and quantum mechanics, which have long been at odds.
“This is a needle in a haystack [finding]. And the paper that started this whole thing is from way back in 1993,” says Loren Pfeiffer at Princeton University. He wrote that paper with several colleagues including Aron Pinczuk, who passed away in 2022 before they could find hints of the elusive particles. READ MORE...
Monday, August 5
Quantum Mechanics United with General Relativity
Scientists have revealed a radical theory that seeks to reconcile two pillars of modern physics – quantum mechanics and Einstein’s theory of general relativity. (CREDIT: Isaac Young)
In a cutting-edge development that has sent shockwaves through the scientific community, researchers at University College London (UCL) have unveiled a radical theory that seeks to reconcile two pillars of modern physics – quantum mechanics and Einstein's general theory of relativity.
These two theories, which have been the foundation of physics for over a century, have long been at odds with each other, and their unification has remained an elusive quest.
Today, we dive into the world of quantum gravity, a field of study that aims to bridge the gap between the quantum realm, which governs the behavior of particles at the smallest scales, and the macroscopic world, where gravity shapes the very fabric of spacetime.
While the prevailing consensus has been that Einstein's theory of gravity must be modified to fit within the framework of quantum theory, a new theory, coined as a "postquantum theory of classical gravity," challenges this assumption in a thought-provoking way. READ MORE...
These two theories, which have been the foundation of physics for over a century, have long been at odds with each other, and their unification has remained an elusive quest.
Today, we dive into the world of quantum gravity, a field of study that aims to bridge the gap between the quantum realm, which governs the behavior of particles at the smallest scales, and the macroscopic world, where gravity shapes the very fabric of spacetime.
While the prevailing consensus has been that Einstein's theory of gravity must be modified to fit within the framework of quantum theory, a new theory, coined as a "postquantum theory of classical gravity," challenges this assumption in a thought-provoking way. READ MORE...
Thursday, June 13
Quantum Mechanics in Ultra Cold
There's a hot new BEC in town that has nothing to do with bacon, egg, and cheese. You won't find it at your local bodega, but in the coldest place in New York: the lab of Columbia physicist Sebastian Will, whose experimental group specializes in pushing atoms and molecules to temperatures just fractions of a degree above absolute zero.
Writing in Nature, the Will lab, supported by theoretical collaborator Tijs Karman at Radboud University in the Netherlands, has successfully created a unique quantum state of matter called a Bose-Einstein Condensate (BEC) out of molecules.
Their BEC, cooled to just five nanoKelvin, or about -459.66°F, and stable for a strikingly long two seconds, is made from sodium-cesium molecules. Like water molecules, these molecules are polar, meaning they carry both a positive and a negative charge.
The imbalanced distribution of electric charge facilitates the long-range interactions that make for the most interesting physics, noted Will. READ MORE...
Thursday, June 6
Quantum Physics Simplified
Quantum mechanics is simultaneously our most powerful and weirdest scientific theory. It’s powerful because it offers exquisite control over the nanoworld of molecular, atomic, and subatomic phenomena. It’s weird because, while we have a complete mathematical formalism, we physicists have been arguing for more than a century over what that formalism means. In other words, unlike other physical theories, the mathematics of quantum mechanics has no clear interpretation. That means physicists and philosophers have been left arguing about which interpretation makes the most sense. Sometimes the idea of “simplicity” is invoked to answer that question.
The “simplest” explanation
There are two main parts of the quantum formalism. The first is what’s called the dynamical equation. This part gives us a mathematical description of how undisturbed systems evolve. We physicists love our dynamical equations — things like Newton’s equations for particles or Maxwell’s equations for electromagnetic waves. In classical physics, the dynamical equation was pretty much the end of the story. Nothing else was required and we came to think of those equations as existing “out there.” They were timeless laws of physics that never required any reference to what physicists were doing. READ MORE...
Wednesday, June 5
The Quantum Universe
Arrows of Time
As far as we know, the fundamental dynamical laws are time neutral --- preferring no direction of time over another. Yet our universe exhibits a number of `arrows of time' --- general phenomena that distinguish directions in time. There is the thermodynamic arrow of time --- the fact that presently isolated systems are mostly evolving towards equilibrium in the same direction of time. There is the electromagnetic arrow of time --- electromagnetic radiation is retarded.
There is the psychological arrow of time --- we remember the past, experience the present, and predict the future. There are the arrows of time supplied by the expansion of the universe and the growth of inhomogeneity. And then, there is the quantum mechanical arrow of time defined in Copenhagen quantum mechanics by the direction in time the wave function of a subsystem is reduced on measurement. The papers below in various ways show how arrows of time arise in quantum cosmology from asymmetries in quantum conditions that specify our universe even though the dynamical laws are time neutral. READ MORE...
Saturday, May 25
Beyond Hydrogen
Recent discoveries in quantum physics have revealed simpler atomic structures than hydrogen, involving pure electromagnetic interactions between particles like electrons and their antiparticles.
This advancement has significant implications for our understanding of quantum mechanics and fundamental physics, highlighted by new methods for detecting tauonium, which could revolutionize measurements of particle physics.
The hydrogen atom was once considered the simplest atom in nature, composed of a structureless electron and a structured proton. However, as research progressed, scientists discovered a simpler type of atom, consisting of structureless electrons (e-), muons (μ-), or tauons (τ-) and their equally structureless antiparticles.
The hydrogen atom was once considered the simplest atom in nature, composed of a structureless electron and a structured proton. However, as research progressed, scientists discovered a simpler type of atom, consisting of structureless electrons (e-), muons (μ-), or tauons (τ-) and their equally structureless antiparticles.
These atoms are bound together solely by electromagnetic interactions, with simpler structures than hydrogen atoms, providing a new perspective on scientific problems such as quantum mechanics, fundamental symmetry, and gravity. READ MORE...
Wednesday, May 1
Atoms Morph into Quantum Waves
In the 1920s, the pioneering physicist Erwin Schrödinger formulated an equation that fundamentally transformed our understanding of the universe. Schrödinger's equation describes how particles can behave like waves, a concept that underpins much of quantum mechanics.
Now, nearly a century later, researchers have made a remarkable advancement that perfectly recreates Schrödinger's predictions in the laboratory: capturing single atoms morphing into quantum waves.
A Historic Moment in Quantum Imaging
The recent breakthrough involves capturing images of individual atoms exhibiting wave-like behavior. This is a historic achievement, as it provides the clearest image ever seen of atoms behaving like quantum waves, just as predicted by Schrödinger's equation.
A Historic Moment in Quantum Imaging
The recent breakthrough involves capturing images of individual atoms exhibiting wave-like behavior. This is a historic achievement, as it provides the clearest image ever seen of atoms behaving like quantum waves, just as predicted by Schrödinger's equation.
This discovery opens up exciting possibilities for studying and understanding the exotic and often mysterious behavior of atoms at the quantum level. READ MORE...
Sunday, December 10
Theory Unites Gravity and Quantum Mechanics
Modern physics is founded upon two pillars: quantum theory on the one hand, which governs the smallest particles in the universe, and Einstein's theory of general relativity on the other, which explains gravity through the bending of spacetime. But these two theories are in contradiction with each other and a reconciliation has remained elusive for over a century.
The prevailing assumption has been that Einstein's theory of gravity must be modified, or "quantized," in order to fit within quantum theory. This is the approach of two leading candidates for a quantum theory of gravity, string theory and loop quantum gravity. READ MORE...
Friday, July 14
Time Reversal
A team of researchers from the University of Twente has successfully illustrated that quantum mechanics and thermodynamics can coexist by using an optical chip with photon channels. The channels individually showed disorder in line with thermodynamics, while the overall system complied with quantum mechanics due to the entanglement of subsystems, proving that information can be preserved and transferred. Credit: University of Twente
It seems quantum mechanics and thermodynamics cannot be true simultaneously. In a new publication, University of Twente researchers use photons in an optical chip to demonstrate how both theories can be true at the same time.
In quantum mechanics, time can be reversed and information is always preserved. That is, one can always find back the previous state of particles. It was long unknown how this could be true at the same time as thermodynamics.
There, time has a direction and information can also be lost. “Just think of two photographs that you put in the sun for too long, after a while you can no longer distinguish them,” explains author Jelmer Renema.
There was already a theoretical solution to this quantum puzzle and even an experiment with atoms, but now the University of Twente (UT) researchers have also demonstrated it with photons. “Photons have the advantage that it is quite easy to reverse time with them,” explains Renema.
There was already a theoretical solution to this quantum puzzle and even an experiment with atoms, but now the University of Twente (UT) researchers have also demonstrated it with photons. “Photons have the advantage that it is quite easy to reverse time with them,” explains Renema.
In the experiment, the researchers used an optical chip with channels through which the photons could pass. At first, they could determine exactly how many photons there were in each channel, but after that, the photons shuffled positions. READ MORE...
Tuesday, February 7
Quantum Entanglement: Spacetime is an illusion
This past December, the physics Nobel Prize was awarded for the experimental confirmation of a quantum phenomenon known for more than 80 years: entanglement. As envisioned by Albert Einstein and his collaborators in 1935, quantum objects can be mysteriously correlated even if they are separated by large distances. But as weird as the phenomenon appears, why is such an old idea still worth the most prestigious prize in physics?
Coincidentally, just a few weeks before the new Nobel laureates were honored in Stockholm, a different team of distinguished scientists from Harvard, MIT, Caltech, Fermilab and Google reported that they had run a process on Google’s quantum computer that could be interpreted as a wormhole. Wormholes are tunnels through the universe that can work like a shortcut through space and time and are loved by science fiction fans, and although the tunnel realized in this recent experiment exists only in a 2-dimensional toy universe, it could constitute a breakthrough for future research at the forefront of physics.
But why is entanglement related to space and time? And how can it be important for future physics breakthroughs? Properly understood, entanglement implies that the universe is “monistic”, as philosophers call it, that on the most fundamental level, everything in the universe is part of a single, unified whole. It is a defining property of quantum mechanics that its underlying reality is described in terms of waves, and a monistic universe would require a universal function.
Already decades ago, researchers such as Hugh Everett and Dieter Zeh showed how our daily-life reality can emerge out of such a universal quantum-mechanical description. But only now are researchers such as Leonard Susskind or Sean Carroll developing ideas on how this hidden quantum reality might explain not only matter but also the fabric of space and time.
Entanglement is much more than just another weird quantum phenomenon. It is the acting principle behind both why quantum mechanics merges the world into one and why we experience this fundamental unity as many separate objects. At the same time, entanglement is the reason why we seem to live in a classical reality. It is—quite literally—the glue and creator of worlds.
Entanglement is much more than just another weird quantum phenomenon. It is the acting principle behind both why quantum mechanics merges the world into one and why we experience this fundamental unity as many separate objects. At the same time, entanglement is the reason why we seem to live in a classical reality. It is—quite literally—the glue and creator of worlds.
Entanglement applies to objects comprising two or more components and describes what happens when the quantum principle that “everything that can happen actually happens” is applied to such composed objects. Accordingly, an entangled state is the superposition of all possible combinations that the components of a composed object can be in to produce the same overall result. It is again the wavy nature of the quantum domain that can help to illustrate how entanglement actually works.
Picture a perfectly calm, glassy sea on a windless day. Now ask yourself, how can such a plane be produced by overlaying two individual wave patterns? One possibility is that superimposing two completely flat surfaces results again in a completely level outcome. But another possibility that might produce a flat surface is if two identical wave patterns shifted by half an oscillation cycle were to be superimposed on one another, so that the wave crests of one pattern annihilate the wave troughs of the other one and vice versa. If we just observed the glassy ocean, regarding it as the result of two swells combined, there would be no way for us to find out about the patterns of the individual swells.
Picture a perfectly calm, glassy sea on a windless day. Now ask yourself, how can such a plane be produced by overlaying two individual wave patterns? One possibility is that superimposing two completely flat surfaces results again in a completely level outcome. But another possibility that might produce a flat surface is if two identical wave patterns shifted by half an oscillation cycle were to be superimposed on one another, so that the wave crests of one pattern annihilate the wave troughs of the other one and vice versa. If we just observed the glassy ocean, regarding it as the result of two swells combined, there would be no way for us to find out about the patterns of the individual swells.
What sounds perfectly ordinary when we talk about waves has the most bizarre consequences when applied to competing realities. If your neighbor told you she had two cats, one live cat and a dead one, this would imply that either the first cat or the second one is dead and that the remaining cat, respectively, is alive—it would be a strange and morbid way of describing one’s pets, and you may not know which one of them is the lucky one, but you would get the neighbor’s drift. Not so in the quantum world.
In quantum mechanics, the very same statement implies that the two cats are merged in a superposition of cases, including the first cat being alive and the second one dead and the first cat being dead while the second one lives, but also possibilities where both cats are half alive and half dead, or the first cat is one-third alive, while the second feline adds the missing two-thirds of life. In a quantum pair of cats, the fates and conditions of the individual animals get dissolved entirely in the state of the whole. Likewise, in a quantum universe, there are no individual objects. All that exists is merged into a single “One.” READ MORE...
Tuesday, May 3
Time Does Not Exist (?)
Does time exist? The answer to this question may seem obvious: Of course it does! Just look at a calendar or a clock.
But developments in physics suggest the non-existence of time is an open possibility, and one that we should take seriously.
How can that be, and what would it mean? It'll take a little while to explain, but don't worry: Even if time doesn't exist, our lives will go on as usual.
A crisis in physics
Physics is in crisis. For the past century or so, we have explained the Universe with two wildly successful physical theories: general relativity and quantum mechanics.
Quantum mechanics describes how things work in the incredibly tiny world of particles and particle interactions. General relativity describes the big picture of gravity and how objects move.
Both theories work extremely well in their own right, but the two are thought to conflict with one another. Though the exact nature of the conflict is controversial, scientists generally agree both theories need to be replaced with a new, more general theory.
Physicists want to produce a theory of "quantum gravity" that replaces general relativity and quantum mechanics, while capturing the extraordinary success of both. Such a theory would explain how gravity's big picture works at the miniature scale of particles.
Time in quantum gravity
It turns out that producing a theory of quantum gravity is extraordinarily difficult.
One attempt to overcome the conflict between the two theories is string theory. String theory replaces particles with strings vibrating in as many as 11 dimensions. READ MORE...
It turns out that producing a theory of quantum gravity is extraordinarily difficult.
One attempt to overcome the conflict between the two theories is string theory. String theory replaces particles with strings vibrating in as many as 11 dimensions. READ MORE...
Thursday, April 21
Weirdness of Quantum Mechanics
Quantum mechanics has a way of taking your mind to places it just doesn’t want to go. Famously hard to understand and impossible to intuit, concepts such as quantum entanglement and superposition really make sense only when viewed through a mathematical lens. Plain language most often leads you down dead ends or false paths that end miles away from reality, with carelessly chosen words propagating misunderstandings at the speed of the internet.
A well-known case in point comes from Albert Einstein. The baked-in weirdness of quantum mechanics troubled him, leading to two celebrated quotes. One— “God does not play dice with the universe”—expressed his unease about the reign of probability over certainty in the quantum realm.
In the other quote, Einstein challenged the notion of the probabilistic correlations among particles, known as quantum entanglement, saying, “I cannot seriously believe in it because the theory cannot be reconciled with the idea that physics should represent a reality in time and space, free from spooky action at a distance.”
That last phrase has launched a thousand misguided speculations about faster-than-light communications. The main problem lies in the word action. It leans toward cause and effect—something directly affecting something else—and implies an unknown mechanism instantaneously operating on widely separated particles.
That influence would clearly violate both the locality principle in physics (objects are only influenced by what’s nearby) and Einstein’s own Special Theory of Relativity, which set the universal speed limit at the speed of light, a theory backed up by observational evidence for a hundred years.
Entanglement refers to the condition of a system composed of atomic-scale particles whose states cannot be fully described independently or individually. John Preskill of Caltech described the situation with a literary metaphor. Someone who read 10 pages of a 100-page book composed in the classical, or non-quantum, physics world, would learn 10% of the book.
Entanglement refers to the condition of a system composed of atomic-scale particles whose states cannot be fully described independently or individually. John Preskill of Caltech described the situation with a literary metaphor. Someone who read 10 pages of a 100-page book composed in the classical, or non-quantum, physics world, would learn 10% of the book.
Reading 10 pages of a quantum book would reveal almost nothing about the book’s contents. As Preskill says, “nearly all the information in the book is encoded in the correlations among the pages.” This principle of quantum mechanics has practical application as the basis for the power of quantum computing and other technologies because you can store information globally within the quantum system. READ MORE...
Wednesday, March 16
Quantum Mechanics and Free Will
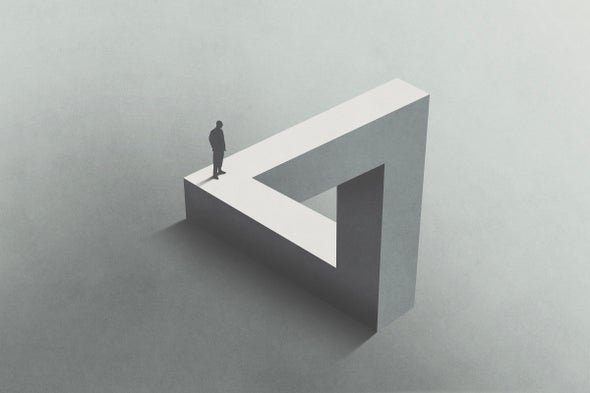
Credit: francescoch/Getty Images
A conjecture called superdeterminism, outlined decades ago, is a response to several peculiarities of quantum mechanics: the apparent randomness of quantum events; their apparent dependence on human observation, or measurement; and the apparent ability of a measurement in one place to determine, instantly, the outcome of a measurement elsewhere, an effect called nonlocality.
Einstein, who derided nonlocality as “spooky action at a distance,” insisted that quantum mechanics must be incomplete; there must be hidden variables that the theory overlooks. Superdeterminism is a radical hidden-variables theory proposed by physicist John Bell. He is renowned for a 1964 theorem, now named after him, that dramatically exposes the nonlocality of quantum mechanics.
Bell said in a BBC interview in 1985 that the puzzle of nonlocality vanishes if you assume that “the world is superdeterministic, with not just inanimate nature running on behind-the-scenes clockwork, but with our behavior, including our belief that we are free to choose to do one experiment rather than another, absolutely predetermined.”
In a recent video, physicist Sabine Hossenfelder, whose work I admire, notes that superdeterminism eliminates the apparent randomness of quantum mechanics. “In quantum mechanics,” she explains, “we can only predict probabilities for measurement outcomes, rather than the measurement outcomes themselves. The outcomes are not determined, so quantum mechanics is indeterministic. Superdeterminism returns us to determinism.”
“The reason we can’t predict the outcome of a quantum measurement,” she explains, “is that we are missing information,” that is, hidden variables. Superdeterminism, she notes, gets rid of the measurement problem and nonlocality as well as randomness. Hidden variables determine in advance how physicists carry out the experiments; physicists might think they are choosing one option over another, but they aren’t. Hossenfelder calls free will “logically incoherent nonsense.”
Hossenfelder predicts that physicists might be able to confirm superdeterminism experimentally. “At some point,” she says, “it’ll just become obvious that measurement outcomes are actually much more predictable than quantum mechanics says. Indeed, maybe someone already has the data, they just haven’t analyzed it the right way.” Hossenfelder defends superdeterminism in more detail in a technical paper written with physicist Tim Palmer.
A conjecture called superdeterminism, outlined decades ago, is a response to several peculiarities of quantum mechanics: the apparent randomness of quantum events; their apparent dependence on human observation, or measurement; and the apparent ability of a measurement in one place to determine, instantly, the outcome of a measurement elsewhere, an effect called nonlocality.
Einstein, who derided nonlocality as “spooky action at a distance,” insisted that quantum mechanics must be incomplete; there must be hidden variables that the theory overlooks. Superdeterminism is a radical hidden-variables theory proposed by physicist John Bell. He is renowned for a 1964 theorem, now named after him, that dramatically exposes the nonlocality of quantum mechanics.
Bell said in a BBC interview in 1985 that the puzzle of nonlocality vanishes if you assume that “the world is superdeterministic, with not just inanimate nature running on behind-the-scenes clockwork, but with our behavior, including our belief that we are free to choose to do one experiment rather than another, absolutely predetermined.”
In a recent video, physicist Sabine Hossenfelder, whose work I admire, notes that superdeterminism eliminates the apparent randomness of quantum mechanics. “In quantum mechanics,” she explains, “we can only predict probabilities for measurement outcomes, rather than the measurement outcomes themselves. The outcomes are not determined, so quantum mechanics is indeterministic. Superdeterminism returns us to determinism.”
“The reason we can’t predict the outcome of a quantum measurement,” she explains, “is that we are missing information,” that is, hidden variables. Superdeterminism, she notes, gets rid of the measurement problem and nonlocality as well as randomness. Hidden variables determine in advance how physicists carry out the experiments; physicists might think they are choosing one option over another, but they aren’t. Hossenfelder calls free will “logically incoherent nonsense.”
Hossenfelder predicts that physicists might be able to confirm superdeterminism experimentally. “At some point,” she says, “it’ll just become obvious that measurement outcomes are actually much more predictable than quantum mechanics says. Indeed, maybe someone already has the data, they just haven’t analyzed it the right way.” Hossenfelder defends superdeterminism in more detail in a technical paper written with physicist Tim Palmer.
TO READ MORE, CLICK HERE...
Sunday, August 29
Metaphysical Mysteries
In my 20s, I had a friend who was brilliant, charming, Ivy-educated and rich, heir to a family fortune. I’ll call him Gallagher. He could do anything he wanted. He experimented, dabbling in neuroscience, law, philosophy and other fields.
But he was so critical, so picky, that he never settled on a career. Nothing was good enough for him. He never found love for the same reason. He also disparaged his friends’ choices, so much so that he alienated us. He ended up bitter and alone. At least that’s my guess. I haven’t spoken to Gallagher in decades.
There is such a thing as being too picky, especially when it comes to things like work, love and nourishment (even the pickiest eater has to eat something). That’s the lesson I gleaned from Gallagher. But when it comes to answers to big mysteries, most of us aren’t picky enough.
There is such a thing as being too picky, especially when it comes to things like work, love and nourishment (even the pickiest eater has to eat something). That’s the lesson I gleaned from Gallagher. But when it comes to answers to big mysteries, most of us aren’t picky enough.
We settle on answers for bad reasons, for example, because our parents, priests or professors believe it. We think we need to believe something, but actually we don’t. We can, and should, decide that no answers are good enough. We should be agnostics.
Some people confuse agnosticism (not knowing) with apathy (not caring). Take Francis Collins, a geneticist who directs the National Institutes of Health. He is a devout Christian, who believes that Jesus performed miracles, died for our sins and rose from the dead. In his 2006 bestseller The Language of God,
Some people confuse agnosticism (not knowing) with apathy (not caring). Take Francis Collins, a geneticist who directs the National Institutes of Health. He is a devout Christian, who believes that Jesus performed miracles, died for our sins and rose from the dead. In his 2006 bestseller The Language of God,
Collins calls agnosticism a “cop-out.” When I interviewed him, I told him I am an agnostic and objected to “cop-out.” READ MORE
Thursday, August 5
What is Life
In 1943, one of the fathers of quantum mechanics, famous for his equation and his cat, Erwin Schrödinger, turned his attention to a problem that was seemingly simple but defied an easy answer. As World War 2 raged, he published a book titled What is Life?
Based on a series of lectures given in Dublin, the book’s theme was to answer the question: “how can the events in space and time which take place within the spatial boundary of a living organism be accounted for by physics and chemistry?”
In other words: What is Life? Or, from a physicist’s point of view, how can life arise from inanimate matter.
Much of the lecture discussed the requirement for genetic material and some sort of encoding as well as how life related to thermodynamics — the laws governing energy, heat transport, and disorder.
Although their success largely depended on Rosalind Franklin’s X-ray diffraction experiments, Francis and Crick would also credit Schrödinger’s work for inspiring their research resulting in the discovery of the DNA double helix.
Schrödinger’s primary insight is that life creates order from disorder. In a universe governed by the 2nd law of thermodynamics, that all things tend to maximal disorder, living things maintain small enclaves of order within themselves. Moreover, if you look down to the atomic level, you find that the interiors of living things are extremely chaotic. Heat and molecules diffuse through rapid motion. Everything seems random. Yet the living thing persists, turning all that small scale chaos into large scale order.
Human built machines, by contrast, attempt to maintain order down to the smallest relevant levels. Microchips, for example, depend on orderly transfer of data down to nanometers. Precision machine tools, likewise, function because they have an exact specification at nearly the molecular level. The result is that human tools require careful protection and maintenance and break easily when subjected to the elements.
Life, on the other hand, has withstood the elements for billions of years precisely because it is able to build order out of chaos. READ MORE
Based on a series of lectures given in Dublin, the book’s theme was to answer the question: “how can the events in space and time which take place within the spatial boundary of a living organism be accounted for by physics and chemistry?”
In other words: What is Life? Or, from a physicist’s point of view, how can life arise from inanimate matter.
Much of the lecture discussed the requirement for genetic material and some sort of encoding as well as how life related to thermodynamics — the laws governing energy, heat transport, and disorder.
Although their success largely depended on Rosalind Franklin’s X-ray diffraction experiments, Francis and Crick would also credit Schrödinger’s work for inspiring their research resulting in the discovery of the DNA double helix.
Schrödinger’s primary insight is that life creates order from disorder. In a universe governed by the 2nd law of thermodynamics, that all things tend to maximal disorder, living things maintain small enclaves of order within themselves. Moreover, if you look down to the atomic level, you find that the interiors of living things are extremely chaotic. Heat and molecules diffuse through rapid motion. Everything seems random. Yet the living thing persists, turning all that small scale chaos into large scale order.
Human built machines, by contrast, attempt to maintain order down to the smallest relevant levels. Microchips, for example, depend on orderly transfer of data down to nanometers. Precision machine tools, likewise, function because they have an exact specification at nearly the molecular level. The result is that human tools require careful protection and maintenance and break easily when subjected to the elements.
Life, on the other hand, has withstood the elements for billions of years precisely because it is able to build order out of chaos. READ MORE
Monday, July 26
Consciousness and Quantum Physics
One of the most important open questions in science is how our consciousness is established. In the 1990s, long before winning the 2020 Nobel Prize in Physics for his prediction of black holes, physicist Roger Penrose teamed up with anesthesiologist Stuart Hameroff to propose an ambitious answer.
They claimed that the brain's neuronal system forms an intricate network and that the consciousness this produces should obey the rules of quantum mechanics – the theory that determines how tiny particles like electrons move around. This, they argue, could explain the mysterious complexity of human consciousness.
Penrose and Hameroff were met with incredulity. Quantum mechanical laws are usually only found to apply at very low temperatures. Quantum computers, for example, currently operate at around -272°C. At higher temperatures, classical mechanics takes over.
Since our body works at room temperature, you would expect it to be governed by the classical laws of physics. For this reason, the quantum consciousness theory has been dismissed outright by many scientists – though others are persuaded supporters. READ MORE
They claimed that the brain's neuronal system forms an intricate network and that the consciousness this produces should obey the rules of quantum mechanics – the theory that determines how tiny particles like electrons move around. This, they argue, could explain the mysterious complexity of human consciousness.
Penrose and Hameroff were met with incredulity. Quantum mechanical laws are usually only found to apply at very low temperatures. Quantum computers, for example, currently operate at around -272°C. At higher temperatures, classical mechanics takes over.
Since our body works at room temperature, you would expect it to be governed by the classical laws of physics. For this reason, the quantum consciousness theory has been dismissed outright by many scientists – though others are persuaded supporters. READ MORE
Wednesday, June 2
the Quantum Revolution
But that solidity and independence is a kind illusion or, at least, so says the very physics that lets these words appear on the screen of your computer, smart phone or tablet.
That physics, called quantum mechanics, represents the most powerful theory human beings have ever developed. And while we scientists know how to use it to make all those digital devices, we do not know what it means. We don't know what it's telling us about the fundamental nature of reality. It is into that chasm that acclaimed theoretical physicist and author Carlo Rovelli leaps with his new book Helgoland. Making Sense of the Quantum Revolution.
It's a leap you will want to take with him.
Quantum mechanics is now more than 100 years old. It was born at the turn of the 20th century as physicists probed the atomic and subatomic realms. Much to their chagrin, reality turned out to be a lot weirder at those scales than in the "classical" realm of ordinary experience. The theory they developed to control the objects of their experiments was an intellectual triumph. Almost all of the technological miracles we live with now — from CAT scans to computers — can be traced to that achievement. But quantum mechanics never really "explained" the strangeness of the microworld. It just gave us a method for making incredibly accurate predictions and building stuff. TO READ MORE, CLICK HERE...
Subscribe to:
Posts (Atom)